Polarization-Maintaining Dispersion-Compensating Fiber Optic Patch Cables
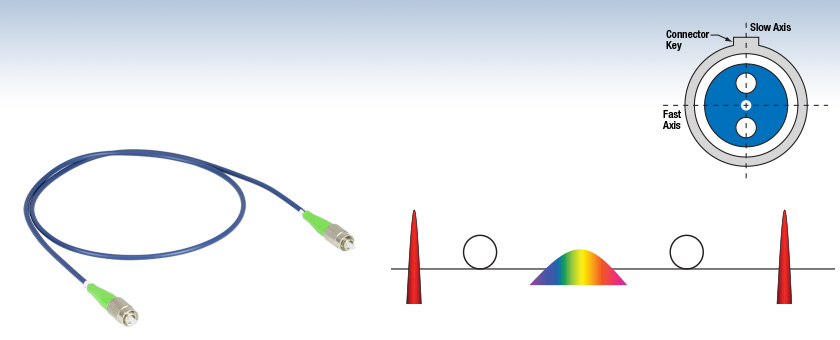
- Operates Between 1510 nm and 1620 nm
- Compensates for Both Dispersion and Dispersion Slope
- Polarization-Maintaining Fiber with FC/APC Connectors on Both Ends
PMDCFA2
Input Pulse
Transmission Fiber
Dispersed Pulse
Dispersion-Compensating Fiber
Compensated Pulse

Please Wait
PM Fiber Patch Cable Selection Guide |
---|
FC/PC to FC/PC |
FC/APC to FC/APC |
FC/PC to FC/APC Hybrid |
High-ER Cables FC/PC, FC/APC, and Hybrid |
AR-Coated FC/PC and Hybrid |
Dispersion-Compensating FC/APC |
HR-Coated FC/PC and FC/APC |
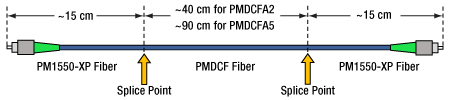
Click to Enlarge
The PMDCF fiber used in these patch cables has a short section of PM1550-XP fiber spliced to each end to minimize loss when connecting to other PM patch cables.
Features
- Dispersion and Dispersion Slope Exactly Compensate 2 m or 5 m Length PM1550-XP Fiber Patch Cables
- Narrow Key (2.0 mm) Aligned to Slow Axis
- Typical Return Loss of 60 dB
- Ceramic 8° Angled Ferrules (APC)
- Ø3 mm Protective Outer Jacket
- Individual Test Report Included with Each Cable;
Click Here for Sample Data Sheet
These polarization-maintaining (PM) fiber optic patch cables incorporate dispersion-compensating fiber (DCF) for applications which require precise control over the dispersion in a system. As shown in the diagram above, each end of the DCF is spliced onto a short length of PM1550-XP fiber to minimize loss when connecting to other PM patch cables. Both ends are teminated with narrow key, ceramic-ferrule FC/APC connectors. These cables feature a high-quality polish, which leads to a typical return loss of 60 dB. Assembled in our facility, each cable is individually tested at 1550 nm to ensure its extinction ratio and insertion loss fall within specifications. Each patch cable is shipped with a data sheet that summarizes the results of this testing (click here for a sample data sheet).
Dispersion-free links can be built by combining a PM DCF cable with a compensated length patch cable that incorporates PM1550-XP fiber. For example, a PMDCFA2 cable can be connected with a P3-1550PM-FC-2 cable to create a dispersion-free fiber link with an approximate length of 2.7 m. Similarly, a PMDCFA5 cable can be connected to a P3-1550PM-FC-5 cable to create a dispersion-free fiber link with an approximate length of 6.2 m. For more information on dispersion in fiber and the use of DCF, see the DCF tab.
Two protective caps are included with each patch cable that shield the ferrule ends from dust and other hazards. Additional CAPF Plastic Fiber Caps and CAPFM Metal Threaded Fiber Caps for FC/APC-terminated ends are also sold separately.
PM patch cables like the ones sold on this page are available in various lengths and tubing. Please contact Tech Support for more information.
Item # | PMDCFA2 | PMDCFA5 |
---|---|---|
Operating Wavelength | 1510 - 1620 nm | |
Cutoff Wavelength | 1400 nm | |
Cable Fiber Typea | PMDCF with Two Short Sections of PM1550-XP Spliced to Each End (PANDA) | |
Cable Length | 0.70 ± 0.05 m | 1.20 ± 0.05 m |
Compensated Fiber | 2 m of PM1550-XP | 5 m of PM1550-XP |
Total Dispersion | -0.034 ± 0.004 ps/nm | -0.085 ± 0.009 ps/nm |
Total Dispersion Slope | -1.1 x 10-4 ± 0.1 x 10-4 ps/nm2 | -2.8 x 10-4 ± 0.2 x 10-4 ps/nm2 |
Insertion Lossb | <2.5 dB | |
Extinction Ratiob | >19 dB | |
Optical Return Lossb | 60 dB (Typical) | |
Connector Type | FC/APC | |
Key Width | 2.00 mm ± 0.02 | |
Key Alignment Type | Narrow Key Aligned to Slow Axis | |
Jacket Type | FT030-BLUE | |
Operating Temperature | 0 to 70 °C | |
Storage Temperature | -45 to 85 °C |
Dispersion in Optical Fiber
Chromatic dispersion, D, in an optical fiber occurs when the group velocity and phase velocity of an optical pulse depend on the optical wavelength/frequency. It is primarily the sum of two components, material dispersion and waveguide dispersion:
Material dispersion arises from the change in a material's refractive index with wavelength, which changes the propagation velocity of light as a function of wavelength. Waveguide dispersion is a separate effect, arising from the geometry of the fiber optic waveguide. Waveguide properties are also a function of wavelength; consequently, changing the wavelength affects how light is guided in a single-mode fiber. For example, decreasing the wavelength will increase the relative waveguide dimensions, causing a change in the distribution of light in the cladding and core.
Another useful parameter is the dispersion coefficient, β, which is also called the phase constant or mode-propagation constant when featured in the nonlinear Schrodinger equation. If the optical pulse propagates along a fiber of length, L, then the associated phase shift is defined as:Where dDfiber/dλ is known as the dispersion slope, which can be positive, negative, or zero, and written as:
Group velocity dispersion (GVD) is the temporal pulse broadening due to different group velocities, and it has significant influence on optical pulse widths on the order of picoseconds or shorter. The group velocity, vg, can be defined as the rate at which the entire pulse envelope will propagate:
Which allows the group velocity dispersion to be defined as:
There is no change in the shape of the temporal pulse when GVD equals zero, however there will always be temporal broadening when GVD is nonzero. When the GVD is greater than zero, the longer wavelength components will propagate faster than the shorter wavelengths; and when the GVD is less than zero, the longer wavelength components will propagate more slowly.
Polarization-mode dispersion (PMD) in typical single-mode fiber occurs as a result of birefringence in the fiber due to asymmetries in fiber stress and geometry. In the frequency domain, it presents itself as a linear change in a fixed input polarization with respect to frequency. In the time domain, it presents itself as the mean time delay of a pulse propagating along the fiber. The group delay is the difference between the mean arrival times at the fiber input and the fiber output.
Polarization-state pairs (PSP) are orthogonal pairs of polarization states at the optical fiber input. For polarization-maintaining fibers, these are the fiber’s fast and slow axes, which are treated separately and generally have different phase shifts and group delays. The differential group delay (DGD) is the difference in group delay between the orthogonal pairs of polarization states. DGD increases proportionally to the square root of the fiber length. Polarization-mode dispersion can be defined as a vector that has a magnitude equal to the DGD and points in the direction of the slow axis.
Dispersion-Compensating Fiber
Since dispersion is inevitable in optical fibers, dispersion-compensating fibers (DCF) can be incorporated into optical systems. The overall dispersion of these fibers is opposite in sign and much larger in magnitude than that of standard fiber, so they can be used to cancel out or compensate for the dispersion of a standard single-mode or polarization-maintaining fiber. A negative dispersion slope enables effective cancellation of dispersion over a larger wavelength range, since the dispersion slope of standard fiber is usually positive. Generally, a short length of DCF is spliced into a longer length of standard fiber to compensate for dispersion, as shown in the example below.
The dispersion-compensating fiber should be selected to match the dispersion of a regular SM or PM fiber, not only at a single wavelength, but over the whole spectral range of the optical pulse. This means that the DCF should match not only the dispersion, D, but the dispersion slope, dDfiber/Dλ. The ratio of these two factors is called the relative dispersion slope. Similarly, the ratio β2/β3 can be used as another numerical parameter to optimize fiber selection. The more similar these parameters are for the DCF and the standard fiber, the less distorted and impaired the transmitted optical pulse will be at the spliced fibers’ output.
To determine the optimal length for a DCF using these matching conditions, one can solve the following coupled equations using the dispersion parameters at the selected wavelength:
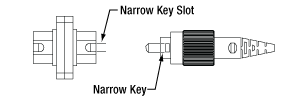
Click to Enlarge
Mating Between a Narrow-Key Mating Sleeve and Connector
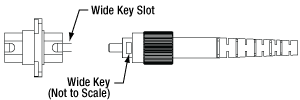
Click to Enlarge
Mating Between a Wide-Key Mating Sleeve and Connector
FC/PC and FC/APC Patch Cable Key Alignment
FC/PC and FC/APC Patch Cables are equipped with either a 2.0 mm narrow or 2.2 mm wide alignment key that fits into a corresponding slot on a mated component. These keys and slots are essential to correctly align the cores of connected fiber patch cables and minimize the insertion loss of the connection.
As an example, Thorlabs designs and manufactures mating sleeves for FC/PC- and FC/APC-terminated patch cables to precise specifications that ensure good alignment when used correctly. To ensure the best alignment, the alignment key on the patch cable is inserted into the corresponding narrow or wide-key slot on the mating sleeve.
Wide-Key-Slot Mating Sleeves
2.2 mm wide-key-slot mating sleeves are compatible with both wide-key and narrow-key connectors. However, using a narrow-key connector in a wide-key slot will allow the connector to rotate slightly in the mating sleeve (as shown in the animation below and to the left). While this configuration is acceptable for patch cables with FC/PC connectors, for FC/APC applications, we recommend using narrow-key-slot mating sleeves to ensure optimum alignment.
Narrow-Key-Slot Mating Sleeves
2.0 mm narrow-key-slot mating sleeves allow for optimal alignment of angled, narrow-key FC/APC connectors, as shown in the animation below and to the right. Therefore, they are not compatible with connectors that have a 2.2 mm wide key. Please note that all FC/PC and FC/APC patch cables manufactured by Thorlabs use narrow key connectors.
Once a narrow key connector is inserted into a narrow-key-slot mating sleeve, the connector will not rotate. We therefore recommend these mating sleeves for FC/PC and FC/APC connectors with narrow keys.
When a narrow key connector is inserted into a wide-key-slot mating sleeve, the connector has room to rotate. For narrow key FC/PC connectors, this is acceptable, but for narrow key FC/APC connectors, significant coupling losses will result.
Quick Links |
---|
Damage at the Air / Glass Interface |
Intrinsic Damage Threshold |
Preparation and Handling of Optical Fibers |
Laser-Induced Damage in Silica Optical Fibers
The following tutorial details damage mechanisms relevant to unterminated (bare) fiber, terminated optical fiber, and other fiber components from laser light sources. These mechanisms include damage that occurs at the air / glass interface (when free-space coupling or when using connectors) and in the optical fiber itself. A fiber component, such as a bare fiber, patch cable, or fused coupler, may have multiple potential avenues for damage (e.g., connectors, fiber end faces, and the device itself). The maximum power that a fiber can handle will always be limited by the lowest limit of any of these damage mechanisms.
While the damage threshold can be estimated using scaling relations and general rules, absolute damage thresholds in optical fibers are very application dependent and user specific. Users can use this guide to estimate a safe power level that minimizes the risk of damage. Following all appropriate preparation and handling guidelines, users should be able to operate a fiber component up to the specified maximum power level; if no maximum is specified for a component, users should abide by the "practical safe level" described below for safe operation of the component. Factors that can reduce power handling and cause damage to a fiber component include, but are not limited to, misalignment during fiber coupling, contamination of the fiber end face, or imperfections in the fiber itself. For further discussion about an optical fiber’s power handling abilities for a specific application, please contact Thorlabs’ Tech Support.
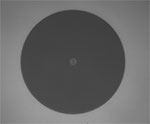
Click to Enlarge
Undamaged Fiber End
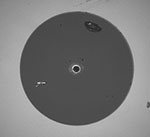
Click to Enlarge
Damaged Fiber End
Damage at the Air / Glass Interface
There are several potential damage mechanisms that can occur at the air / glass interface. Light is incident on this interface when free-space coupling or when two fibers are mated using optical connectors. High-intensity light can damage the end face leading to reduced power handling and permanent damage to the fiber. For fibers terminated with optical connectors where the connectors are fixed to the fiber ends using epoxy, the heat generated by high-intensity light can burn the epoxy and leave residues on the fiber facet directly in the beam path.
Estimated Optical Power Densities on Air / Glass Interfacea | ||
---|---|---|
Type | Theoretical Damage Thresholdb | Practical Safe Levelc |
CW (Average Power) |
~1 MW/cm2 | ~250 kW/cm2 |
10 ns Pulsed (Peak Power) |
~5 GW/cm2 | ~1 GW/cm2 |
Damage Mechanisms on the Bare Fiber End Face
Damage mechanisms on a fiber end face can be modeled similarly to bulk optics, and industry-standard damage thresholds for UV Fused Silica substrates can be applied to silica-based fiber. However, unlike bulk optics, the relevant surface areas and beam diameters involved at the air / glass interface of an optical fiber are very small, particularly for coupling into single mode (SM) fiber. therefore, for a given power density, the power incident on the fiber needs to be lower for a smaller beam diameter.
The table to the right lists two thresholds for optical power densities: a theoretical damage threshold and a "practical safe level". In general, the theoretical damage threshold represents the estimated maximum power density that can be incident on the fiber end face without risking damage with very good fiber end face and coupling conditions. The "practical safe level" power density represents minimal risk of fiber damage. Operating a fiber or component beyond the practical safe level is possible, but users must follow the appropriate handling instructions and verify performance at low powers prior to use.
Calculating the Effective Area for Single Mode Fibers
The effective area for single mode (SM) fiber is defined by the mode field diameter (MFD), which is the cross-sectional area through which light propagates in the fiber; this area includes the fiber core and also a portion of the cladding. To achieve good efficiency when coupling into a single mode fiber, the diameter of the input beam must match the MFD of the fiber.
As an example, SM400 single mode fiber has a mode field diameter (MFD) of ~Ø3 µm operating at 400 nm, while the MFD for SMF-28 Ultra single mode fiber operating at 1550 nm is Ø10.5 µm. The effective area for these fibers can be calculated as follows:
SM400 Fiber: Area = Pi x (MFD/2)2 = Pi x (1.5 µm)2 = 7.07 µm2 = 7.07 x 10-8 cm2
SMF-28 Ultra Fiber: Area = Pi x (MFD/2)2 = Pi x (5.25 µm)2 = 86.6 µm2 = 8.66 x 10-7 cm2
To estimate the power level that a fiber facet can handle, the power density is multiplied by the effective area. Please note that this calculation assumes a uniform intensity profile, but most laser beams exhibit a Gaussian-like shape within single mode fiber, resulting in a higher power density at the center of the beam compared to the edges. Therefore, these calculations will slightly overestimate the power corresponding to the damage threshold or the practical safe level. Using the estimated power densities assuming a CW light source, we can determine the corresponding power levels as:
SM400 Fiber: 7.07 x 10-8 cm2 x 1 MW/cm2 = 7.1 x 10-8 MW = 71 mW (Theoretical Damage Threshold)
7.07 x 10-8 cm2 x 250 kW/cm2 = 1.8 x 10-5 kW = 18 mW (Practical Safe Level)
SMF-28 Ultra Fiber: 8.66 x 10-7 cm2 x 1 MW/cm2 = 8.7 x 10-7 MW = 870 mW (Theoretical Damage Threshold)
8.66 x 10-7 cm2 x 250 kW/cm2 = 2.1 x 10-4 kW = 210 mW (Practical Safe Level)
Effective Area of Multimode Fibers
The effective area of a multimode (MM) fiber is defined by the core diameter, which is typically far larger than the MFD of an SM fiber. For optimal coupling, Thorlabs recommends focusing a beam to a spot roughly 70 - 80% of the core diameter. The larger effective area of MM fibers lowers the power density on the fiber end face, allowing higher optical powers (typically on the order of kilowatts) to be coupled into multimode fiber without damage.
Damage Mechanisms Related to Ferrule / Connector Termination
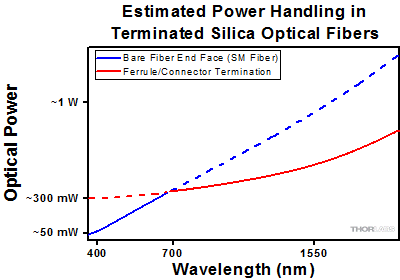
Plot showing approximate input power that can be incident on a single mode silica optical fiber with a termination. Each line shows the estimated power level due to a specific damage mechanism. The maximum power handling is limited by the lowest power level from all relevant damage mechanisms (indicated by a solid line).
Fibers terminated with optical connectors have additional power handling considerations. Fiber is typically terminated using epoxy to bond the fiber to a ceramic or steel ferrule. When light is coupled into the fiber through a connector, light that does not enter the core and propagate down the fiber is scattered into the outer layers of the fiber, into the ferrule, and the epoxy used to hold the fiber in the ferrule. If the light is intense enough, it can burn the epoxy, causing it to vaporize and deposit a residue on the face of the connector. This results in localized absorption sites on the fiber end face that reduce coupling efficiency and increase scattering, causing further damage.
For several reasons, epoxy-related damage is dependent on the wavelength. In general, light scatters more strongly at short wavelengths than at longer wavelengths. Misalignment when coupling is also more likely due to the small MFD of short-wavelength SM fiber that also produces more scattered light.
To minimize the risk of burning the epoxy, fiber connectors can be constructed to have an epoxy-free air gap between the optical fiber and ferrule near the fiber end face. Our high-power multimode fiber patch cables use connectors with this design feature.
Determining Power Handling with Multiple Damage Mechanisms
When fiber cables or components have multiple avenues for damage (e.g., fiber patch cables), the maximum power handling is always limited by the lowest damage threshold that is relevant to the fiber component. In general, this represents the highest input power that can be incident on the patch cable end face and not the coupled output power.
As an illustrative example, the graph to the right shows an estimate of the power handling limitations of a single mode fiber patch cable due to damage to the fiber end face and damage via an optical connector. The total input power handling of a terminated fiber at a given wavelength is limited by the lower of the two limitations at any given wavelength (indicated by the solid lines). A single mode fiber operating at around 488 nm is primarily limited by damage to the fiber end face (blue solid line), but fibers operating at 1550 nm are limited by damage to the optical connector (red solid line).
In the case of a multimode fiber, the effective mode area is defined by the core diameter, which is larger than the effective mode area for SM fiber. This results in a lower power density on the fiber end face and allows higher optical powers (on the order of kilowatts) to be coupled into the fiber without damage (not shown in graph). However, the damage limit of the ferrule / connector termination remains unchanged and as a result, the maximum power handling for a multimode fiber is limited by the ferrule and connector termination.
Please note that these are rough estimates of power levels where damage is very unlikely with proper handling and alignment procedures. It is worth noting that optical fibers are frequently used at power levels above those described here. However, these applications typically require expert users and testing at lower powers first to minimize risk of damage. Even still, optical fiber components should be considered a consumable lab supply if used at high power levels.
Intrinsic Damage Threshold
In addition to damage mechanisms at the air / glass interface, optical fibers also display power handling limitations due to damage mechanisms within the optical fiber itself. These limitations will affect all fiber components as they are intrinsic to the fiber itself. Two categories of damage within the fiber are damage from bend losses and damage from photodarkening.
Bend Losses
Bend losses occur when a fiber is bent to a point where light traveling in the core is incident on the core/cladding interface at an angle higher than the critical angle, making total internal reflection impossible. Under these circumstances, light escapes the fiber, often in a localized area. The light escaping the fiber typically has a high power density, which burns the fiber coating as well as any surrounding furcation tubing.
A special category of optical fiber, called double-clad fiber, can reduce the risk of bend-loss damage by allowing the fiber’s cladding (2nd layer) to also function as a waveguide in addition to the core. By making the critical angle of the cladding/coating interface higher than the critical angle of the core/clad interface, light that escapes the core is loosely confined within the cladding. It will then leak out over a distance of centimeters or meters instead of at one localized spot within the fiber, minimizing the risk of damage. Thorlabs manufactures and sells 0.22 NA double-clad multimode fiber, which boasts very high, megawatt range power handling.
Photodarkening
A second damage mechanism, called photodarkening or solarization, can occur in fibers used with ultraviolet or short-wavelength visible light, particularly those with germanium-doped cores. Fibers used at these wavelengths will experience increased attenuation over time. The mechanism that causes photodarkening is largely unknown, but several fiber designs have been developed to mitigate it. For example, fibers with a very low hydroxyl ion (OH) content have been found to resist photodarkening and using other dopants, such as fluorine, can also reduce photodarkening.
Even with the above strategies in place, all fibers eventually experience photodarkening when used with UV or short-wavelength light, and thus, fibers used at these wavelengths should be considered consumables.
Preparation and Handling of Optical Fibers
General Cleaning and Operation Guidelines
These general cleaning and operation guidelines are recommended for all fiber optic products. Users should still follow specific guidelines for an individual product as outlined in the support documentation or manual. Damage threshold calculations only apply when all appropriate cleaning and handling procedures are followed.
-
All light sources should be turned off prior to installing or integrating optical fibers (terminated or bare). This ensures that focused beams of light are not incident on fragile parts of the connector or fiber, which can possibly cause damage.
-
The power-handling capability of an optical fiber is directly linked to the quality of the fiber/connector end face. Always inspect the fiber end prior to connecting the fiber to an optical system. The fiber end face should be clean and clear of dirt and other contaminants that can cause scattering of coupled light. Bare fiber should be cleaved prior to use and users should inspect the fiber end to ensure a good quality cleave is achieved.
-
If an optical fiber is to be spliced into the optical system, users should first verify that the splice is of good quality at a low optical power prior to high-power use. Poor splice quality may increase light scattering at the splice interface, which can be a source of fiber damage.
-
Users should use low power when aligning the system and optimizing coupling; this minimizes exposure of other parts of the fiber (other than the core) to light. Damage from scattered light can occur if a high power beam is focused on the cladding, coating, or connector.
Tips for Using Fiber at Higher Optical Power
Optical fibers and fiber components should generally be operated within safe power level limits, but under ideal conditions (very good optical alignment and very clean optical end faces), the power handling of a fiber component may be increased. Users must verify the performance and stability of a fiber component within their system prior to increasing input or output power and follow all necessary safety and operation instructions. The tips below are useful suggestions when considering increasing optical power in an optical fiber or component.
-
Splicing a fiber component into a system using a fiber splicer can increase power handling as it minimizes possibility of air/fiber interface damage. Users should follow all appropriate guidelines to prepare and make a high-quality fiber splice. Poor splices can lead to scattering or regions of highly localized heat at the splice interface that can damage the fiber.
-
After connecting the fiber or component, the system should be tested and aligned using a light source at low power. The system power can be ramped up slowly to the desired output power while periodically verifying all components are properly aligned and that coupling efficiency is not changing with respect to optical launch power.
-
Bend losses that result from sharply bending a fiber can cause light to leak from the fiber in the stressed area. When operating at high power, the localized heating that can occur when a large amount of light escapes a small localized area (the stressed region) can damage the fiber. Avoid disturbing or accidently bending fibers during operation to minimize bend losses.
-
Users should always choose the appropriate optical fiber for a given application. For example, large-mode-area fibers are a good alternative to standard single mode fibers in high-power applications as they provide good beam quality with a larger MFD, decreasing the power density on the air/fiber interface.
-
Step-index silica single mode fibers are normally not used for ultraviolet light or high-peak-power pulsed applications due to the high spatial power densities associated with these applications.
Posted Comments: | |
Cristina Amaya Mendez
 (posted 2023-07-21 16:14:01.757) Dear Thorlabs team,
I would like to make an inquiry on the PM DCF optic patch cable. Do you also sell custom lengths? I would need a patched cable with ~3.5 meters of PM-DCF fiber, spliced with PM fibers on both sides to avoid loses.
Could you please tell me if you can fabricate such cable? and if this is the case, what would be an approximate price and lead time?
Thank you in advance! cdolbashian
 (posted 2023-07-27 11:41:41.0) Thank you for reaching out to us with this inquiry! This is potentially something we can do for you as a custom. I have put you in contact with your local Solutions Team, who will assist in facilitating this custom order. |

Item # | Cable Fiber Typea | Cable Length | Operating Wavelength |
Cutoff Wavelength |
Extinction Ratio |
Insertion Lossb |
Total Dispersion | Compensated Fiber Type |
Compensated Length |
---|---|---|---|---|---|---|---|---|---|
PMDCFA2 | PMDCF (PANDA) | 0.70 m | 1510 - 1620 nm | 1400 nm | >19 dB | <2.5 dB | -0.034 ± 0.004 ps/nm | PM1550-XP (PANDA) | 2 m |
PMDCFA5 | 1.20 m | -0.085 ± 0.009 ps/nm | 5 m |