Balanced Amplified Photodetectors with Fast Monitor Output

- Models Available with Bandwidths Up to 2.5 GHz
- Noise Cancellation by Subtraction of 2 Input Signals
- >20 dB Common Mode Rejection Ratio
- Fast Monitor Outputs
PDB415A
Fixed Gain, 100 MHz, Si
Optical Inputs Shown
PDB450C
Switchable Gain, InGaAs
Optical Inputs Shown
PDB482C-AC
Fixed Gain, 2.5 GHz, InGaAs, AC Coupled
Electrical Outputs Shown

Please Wait
Balanced Detector Selection Guide |
---|
Balanced Detectors with Fast Monitor Output |
Large-Area Balanced Amplified Detectors |
Suitable for OCT |
Compact Balanced Amplified Detectors |
Compact Balanced Detectors |
OCT Balanced Detectors with Fast Monitor Output |
Auto-Balanced Detector with Avalanche Photodiodes |
Polarization-Dependent Balanced Detector |
Typical Applications
- Spectroscopy
- Heterodyne Detection
(See Doppler LIDAR Tab) - Optical Coherence Tomography OCT
- Optical Delay Measurements
- THz Detection
Balanced Detector with Fast Monitor Output | |||
---|---|---|---|
Bandwidth | Available Wavelength Ranges |
Fast Monitor Output Bandwidth |
Gain |
Switchable (DC - 0.1, 0.3, 4, 45, or 150 MHz) |
320 to 1000 nm or 800 - 1700 nm |
DC - 1 MHz | Switchable |
DC - 15 MHz | Fixed | ||
DC - 75 MHz | |||
DC - 100 MHz | |||
DC - 200 MHz | |||
DC - 350 MHz | |||
DC - 400 MHz | Optimized for 1060 nm (900 - 1400 nm Range) or Optimized for 1300 nm (1200 - 1700 nm Range) |
DC - 3 MHz | |
DC - 500 MHz | 800 - 1700 nm | DC - 1 MHz | |
30 kHz - 1.0 GHz | Optimized for 1060 nm (900 - 1400 nm Range) |
DC - 3 MHz | |
30 kHz - 1.6 GHz | Optimized for 1300 nm (1200 - 1700 nm Range) |
DC - 3 MHz | |
1 MHz - 2.5 GHz | Optimized for 1060 nm (900 - 1400 nm Range) |
DC - 2.5 MHz |
Features
- Four Wavelength Ranges Available
- 320 - 1000 nm
- 800 - 1700 nm
- 900 - 1400 nm (Optimized for 1060 nm)
- 1200 - 1700 nm (Optimized for 1300 nm)
- >20 dB Common Mode Rejection Ratio
- Fast Monitor Outputs
- Choose a Si or InGaAs Detector
- FC Fiber Inputs Available in All Versions
- Free-Space Inputs Available on Some Detectors (See Below for Details)
- Switchable Power Supply Included
- Switchable Transimpedance Gain Version Available
These balanced photodetectors act as a balanced receiver by subtracting the two optical input signals from each other, resulting in the cancellation of common mode noise. This allows small changes in the signal path to be extracted from the interfering noise floor.
The detectors consist of two balanced photodiodes and an ultra-low-noise, high-speed transimpedance amplifier. The two photodiodes are matched to achieve an excellent common mode rejection ratio (CMRR), leading to better noise reduction. Please see the Operation tab for more details. All of these detectors incorporate three female SMA electrical ports for reduced noise. In addition to the RF-output from the transimpedance amplifier, the Monitor+ and Monitor- ports allow the response of each photodiode to be observed individually.
To block the CW component (the unmodulated part) of the optical input signal, an AC-coupled version of each detector is offered. Note that the PDB480C-AC, PDB481C-AC, and PDB482C-AC are only available AC coupled. This improves the ability to measure a comparably weak, frequency-modulated signal over a strong CW background signal that could saturate the amplifier. The lower cut-off frequency for the AC-coupled versions, with exception to the PDB480C-AC, PDB481C-AC, PDB482C-AC, and PDB835-AC, is guaranteed to be ≤100 Hz, but will typically be below 5 Hz.
Bandwidths up to 350 MHz
Detectors with bandwidths of DC - 350 MHz or lower are available with either Si or InGaAs photodiodes for the 320 - 1000 nm or 800 - 1700 nm range, respectively. The inputs on these detectors are not fiber coupled to the photodiodes and come with two removable FC input connectors, making them suitable for either free-space or fiber applications. Although the PDB465 and PDB435 detectors also contain non-fiber-coupled sensors, the FC/PC adapters at the inputs cannot be removed.
Housed in a shielded aluminum enclosure measuring 85 mm x 80 mm x 30 mm, these detectors are post mountable using the included adapter plate, which can be attached to the bottom or side of the housing with the included M2 screws.
Bandwidths of 400 MHz and Higher (Excluding PDB835 Series)
Detectors with bandwidths of DC - 400 MHz or higher other than PDB835 series detectors feature fiber-coupled photodiodes connected to FC/APC optical inputs with exactly length-matched SMF-28e+ or HI1060 fiber to achieve excellent CMRR values across the full detector bandwidth. All of these detectors incorporate InGaAs photodiodes and are optimized for either 1060 nm (900 - 1400 nm range) or 1300 nm (1200 - 1700 nm range). The fiber-coupled design suppresses line artifacts in the OCT image, which generally occur when detector coupling optics are used.
Our highest bandwidth balanced detectors (PDB480C-AC, PDB481C-AC, and PDB482C-AC) are offered in an AC version only. They offer bandwidths up to 2.5 GHz, leading to considerable speed improvements. The ultra-low-distortion output stage supports up to a 2 Vp-p A/D card input range, which, combined with the fiber-coupled design, improves the image quality for OCT applications considerably.
Housed in a shielded aluminum enclosure measuring 85 mm x 80 mm x 30 mm, these detectors are post mountable using the included adapter plate, which can be attached to the bottom or side of the housing with the included M2 screws.
PDB835 Series Ultra-Low-Noise, Artifact-Free Detectors (DC - 500 MHz)
The PDB835C and PDB35C-AC detectors feature free-space InGaAs photodiodes connected to FC/APC optical inputs for the 800 - 1700 nm range. Their transimpedance amplifiers feature ultra-low noise over their entire operating bandwidth (up to 500 MHz; see the Specs tab for details on the minimum NEP). These detectors feature FC/APC connectors which are individually aligned to the photodiode and achieve artifact-free operation. Each detector features a compact 76.7 mm x 60.0 mm x 20.0 mm housing with 8-32 (M4 x 0.7) combi-thread mounting holes on the top and bottom sides for universal mounting.
Power Supply
Each detector is powered by the included ±12 V DC power supply, for which a replacement is available below.
Thorlabs also offers Fiber-Based Interferometers, which feature an integrated balanced detector. If you are primarily interested in using our balanced detectors with OCT systems, a condensed list of detectors suitable for this application can be found here.
Item # | PDB415A(-AC) | PDB415C(-AC) | PDB425A(-AC) | PDB425C(-AC) | PDB435A(-AC) | PDB435C(-AC) |
---|---|---|---|---|---|---|
Detector Type | Si/PIN | InGaAs/PIN | Si/PIN | InGaAs/PIN | Si/PIN | InGaAs/PIN |
Wavelength Range | 320 - 1000 nm | 800 - 1700 nm | 320 - 1000 nm | 800 - 1700 nm | 320 - 1000 nm | 800 - 1700 nm |
Max Responsivity (Typical) | 0.53 A/W | 1.0 A/W | 0.53 A/W | 1.0 A/W | 0.50 A/W | 1.0 A/W |
Active Detector Diameter | 0.8 mm | 0.3 mm | 0.8 mm | 0.3 mm | 0.4 mm | 0.15 mm |
Bandwidth (3 dB) | DC - 100 MHz AC Coupled (-AC Suffix): 100 Hz - 100 MHz |
DC - 75 MHz AC Coupled (-AC Suffix): 100 Hz - 75 MHz |
DC - 350 MHz AC Coupled (-AC Suffix): 100 Hz - 350 MHz |
|||
Common Mode Rejection Ratio | >25 dB | >35 dB | >20 dB | |||
Transimpedance Gain a | 50 x 10 3 V/A | 250 x 10 3 V/A | 10 x 10 3 V/A | |||
Minimum NEP b | 12.03 pW/Hz 1/2 (DC - 100 MHz) |
6.99 pW/Hz 1/2 (DC - 100 MHz) |
9.5 pW/Hz 1/2 (DC - 75 MHz) |
5.2 pW/Hz 1/2 (DC - 75 MHz) |
32.3 pW/Hz 1/2 (DC - 350 MHz) |
15.28 pW/Hz 1/2 (DC - 350 MHz) |
RF Output | ||||||
Conversion Gain RF Output a,c | 26.5 x 10 3 V/W | 50 x 10 3 V/W | 133 x 10 3 V/W | 250 x 10 3 V/W | 5 x 10 3 V/W | 10 x 10 3 V/W |
CW Saturation Power RF Output | 135 µW @ 820 nm | 72 µW @ 1550 nm | 27 µW @ 820 nm | 15 µW @ 1550 nm | 720 µW @ 820 nm | 360 µW @ 1550 nm |
Impedance | 50 Ω | |||||
Max Voltage Swing | ±1.8 V for 50 Ω Load ±3.6 V for High-Impedance Load |
|||||
DC Offset | < ±3 mV | |||||
RF-Output Coupling | DC or AC | |||||
Monitor Outputs | ||||||
Conversion Gain Monitor Outputs | 10 V/mW @ 820 nm | 10 V/mW @ 1550 nm | 10 V/mW @ 820 nm | 10 V/mW @ 1550 nm | 10 V/mW @ 820 nm | 10 V/mW @ 1550 nm |
Impedance | 220 Ω | |||||
Max Voltage Swing | 1.55 V for 50 Ω Load 10 V for High-Z Load |
|||||
Bandwidth | DC to 1 MHz | |||||
Voltage Noise | <180 µV RMS | |||||
DC Offset | < ±2 mV | |||||
General | ||||||
Fiber Optical Inputs d | FC/PC or FC/APC (Removable) |
FC/PC or FC/APC (Not Removable) |
||||
Photodiode Damage Threshold | 20 mW | |||||
Electrical Outputs | SMA (Qty. 3) | |||||
Dimensions | 85 mm x 80 mm x 30 mm (3.35" x 3.15" x 1.18") | |||||
Weight | 0.35 kg (without Power Supply) | |||||
Operating Temperature Range e | 0 to 40 °C |
|||||
Storage Temperature Range | -40 to 70 °C | |||||
Included Power Supply f | ±12 V @ 250 mA (100/120/230 VAC, 50 - 60 Hz, Switchable) |
Item # | PDB440A(-AC) | PDB440C(-AC) | PDB450A(-AC) | PDB450C(-AC) | PDB465A(-AC) | PDB465C(-AC) |
---|---|---|---|---|---|---|
Detector Type | Si/PIN | InGaAs/PIN | Si/PIN | InGaAs/PIN | Si/PIN | InGaAs/PIN |
Wavelength Range | 320 - 1000 nm | 800 - 1700 nm | 320 - 1000 nm | 800 - 1700 nm | 320 - 1000 nm | 800 - 1700 nm |
Max Responsivity (Typical) | 0.53 A/W | 1.0 A/W | 0.53 A/W | 1.0 A/W | 0.50 A/W | 1.0 A/W |
Active Detector Diameter | 0.8 mm | 0.3 mm | 0.8 mm | 0.3 mm | 0.8 mm | 0.15 mm |
Bandwidth (3 dB) | DC - 15 MHz AC Coupled (-AC Suffix): 100 Hz - 15 MHz |
DC - 150, 45, 4, 0.3, 0.1 MHz AC Coupled (-AC Suffix): 100 Hz - 150, 45, 4, 0.3, 0.1 MHz |
DC - 200 MHz AC Coupled (-AC Suffix): 100 Hz - 200 MHz |
|||
Common Mode Rejection Ratio | >35 dB | >25 dB | >25 dB | |||
Transimpedance Gain a | 51 x 10 3 V/A | 10 3 , 10 4 , 10 5 , 10 6 , 10 7 V/A | 30 x 10 3 V/A | |||
Minimum NEP b | 6.9 pW/Hz 1/2 (DC - 15 MHz) |
3.9 pW/Hz 1/2 (DC - 15 MHz) |
DC - 0.1 MHz: 1.4 pW/Hz 1/2 DC - 0.3 MHz: 1.1 pW/Hz 1/2 DC - 4.0 MHz: 3.3 pW/Hz 1/2 DC - 45 MHz: 28.9 pW/Hz 1/2 DC - 150 MHz: 123 pW/Hz 1/2 |
DC - 0.1 MHz: 0.7 pW/Hz 1/2 DC - 0.3 MHz: 0.5 pW/Hz 1/2 DC - 4.0 MHz: 1.55 pW/Hz 1/2 DC - 45 MHz: 14.9 pW/Hz 1/2 DC - 150 MHz: 68.6 pW/Hz 1/2 |
22.86 pW/Hz 1/2 (DC - 200 MHz) |
8.52 pW/Hz 1/2 (DC - 200 MHz) |
RF Output | ||||||
Conversion Gain RF Output a,c | 27 x 10 3 V/W | 51 x 10 3 V/W | 0.53 x 10 3 V/W, 0.53 x 10 4 V/W, 0.53 x 10 5 V/W, 0.53 x 10 6 V/W, 0.53 x 10 7 V/W |
10 3 V/W, 10 4 V/W, 10 5 V/W, 10 6 V/W, 10 7 V/W |
16 x 10 3 V/W | 30 x 10 3 V/W |
CW Saturation Power RF Output | 130 µW @ 820 nm | 70 µW @ 1550 nm | 9 mW @ 820 nm | 4.5 mW @ 1550 nm | 225 µW @ 820 nm | 120 µW @ 1550 nm |
Impedance | 50 Ω | |||||
Max Voltage Swing | ±1.8 for 50 Ω Load ±3.6 V for High-Impedance Load |
±4.6 V (10 3 to 10 6 Gain) a ±10 V (10 7 Gain) a |
±1.8 for 50 Ω Load ±3.6 V for High-Impedance Load |
|||
DC Offset | < ±3 mV | < ±15 mV | < ±3 mV | |||
RF-Output Coupling | DC or AC | |||||
Monitor Outputs | ||||||
Conversion Gain Monitor Outputs | 10 V/mW @ 820 nm | 10 V/mW @ 1550 nm | 10 V/mW @ 820 nm | 10 V/mW @ 1550 nm | 10 V/mW @ 820 nm | 10 V/mW @ 1550 nm |
Impedance | 220 Ω | |||||
Max Voltage Swing | 1.55 V for 50 Ω Load 10 V for High-Z Load |
|||||
Bandwidth | DC to 1 MHz | |||||
Voltage Noise | <180 µV RMS | |||||
DC Offset | < ±2 mV | |||||
General | ||||||
Fiber Optical Inputs d | FC/PC or FC/APC (Removable) |
FC/PC or FC/APC (Not Removable) |
||||
Photodiode Damage Threshold | 20 mW | |||||
Electrical Outputs | SMA (Qty. 3) | |||||
Dimensions | 85 mm x 80 mm x 30 mm (3.35" x 3.15" x 1.18") | |||||
Weight | 0.35 kg (without Power Supply) | |||||
Operating Temperature Range e | 0 to 40 °C |
|||||
Storage Temperature Range | -40 to 70 °C | |||||
Included Power Supply f | ±12 V @ 250 mA (100/120/230 VAC, 50 - 60 Hz, Switchable) |
Item # | PDB470C(-AC) a | PDB471C(-AC) a | PDB480C-AC a | PDB481C-AC a | PDB482C-AC a | PDB835C(-AC) a |
---|---|---|---|---|---|---|
Detector | ||||||
Detector Type | InGaAs/PIN | |||||
Optical Inputs | FC/APC | |||||
Internal Coupling Fiber | SMF-28e+ | HI1060 | SMF-28e+ | HI1060 | None | |
Coupling Loss | <0.5 dB (<0.3 dB Typ.) | <1.0 dB (<0.4 dB Typ.) | <0.5 dB (<0.3 dB Typ.) | <1.0 dB (<0.4 dB Typ.) | N/A | |
Operating Wavelength | Optimized for 1300 nm (1200 - 1700 nm Range) |
Optimized for 1060 nm (900 - 1400 nm Range) |
Optimized for 1300 nm (1200 - 1700 nm Range) |
Optimized for 1060 nm (900 - 1400 nm Range) |
800 - 1700 nm | |
Responsivity (Typical) | 0.9 A/W @ 1550 nm | 0.72 A/W @ 1060 nm | 0.9 A/W @ 1550 nm | 0.72 A/W @ 1060 nm | 1.0 A/W @ 1550 nm | |
Active Detector Diameter | 0.075 mm | 0.080 mm | 0.075 mm | 0.08 mm | ||
Optical Back Reflection | <-40 dB | N/A | ||||
Photodiode Damage Threshold |
10 mW | 5 mW | 10 mW | 5 mW | 20 mW | |
RF OUTPUT | ||||||
RF OUTPUT Bandwidth (3 dB) | DC - 400 MHz AC Coupled (-AC Suffix): 100 Hz - 400 MHz |
30 kHz - 1.6 GHz | 30 kHz - 1.0 GHz | 1 MHz - 2.5 GHz | DC - 500 MHz AC Coupled (-AC Suffix): 1 kHz - 500 MHz |
|
Common Mode Rejection Ratio | >25 dB (Typ. >30 dB) | >20 dB (Typ. >25 dB) | >25 dB | |||
RF OUTPUT Transimpedance Gain | 10 x 10 3 V/A b | 16 x 10 3 V/A c | 28 x 10 3 V/A c | 10 x 10 3 V/A d | ||
RF OUTPUT Conversion Gain | 9 x 10 3 V/W @ 1550 nm b |
7.2 x 10 3 V/W @ 1060 nm b |
14.4 x 10 3 V/W @ 1550 nm c |
11.5 x 10 3 V/W @ 1060 nm c |
20 x 10 3 V/W @ 1060 nm c |
10 x 10 3 V/W @ 1550 nm d,e |
RF OUTPUT Power at 1 dB Compression c |
- | +16.5 dBm Min +18 dBm Typ. |
- | |||
RF OUTPUT CW Saturation Power | 420 µW @ 1550 nm | 530 µW @ 1060 nm | See Note Below | 360 µW @ 1550 nm | ||
RF OUTPUT Coupling | DC or AC | AC Coupling Only | DC or AC | |||
RF OUTPUT Impedance | 50 Ω | |||||
Max Voltage Swing | ±1.9 V for 50 Ω Load ±3.8 V for High-Z Load |
See Note Below | ±1.8 V for 50 Ω Load; ±3.6 V (Linear Over 0 to 2.5 V) for High-Z Load |
|||
Minimum NEP f | 8 pW/Hz 1/2 (DC to 100 MHz) |
9.3 pW/Hz 1/2 (30 kHz to 100 MHz) |
9.0 pW/Hz 1/2 (30 kHz to 100 MHz) |
12.0 pW/Hz 1/2 (1 MHz to 100 MHz) |
6.5 pW/Hz 1/2 (DC to 500 MHz) |
|
Overall Output Voltage Noise | <2.0 mV RMS | <9 mV RMS | <6.5 mV RMS | <12 mV RMS | <1.5 mV RMS | |
DC Offset | N/A | < ±5 mV | ||||
MONITOR Outputs | ||||||
MONITOR Output Impedance | 200 Ω | |||||
MONITOR Output Bandwidth (3 dB) | DC - 3 MHz | DC - 2.5 MHz | DC - 1 MHz | |||
MONITOR Output Conversion Gain, High Z Load b |
9 V/mW @ 1550 nm | 7.2 V/mW @ 1060 nm | 9 V/mW @ 1550 nm | 7.2 V/mW @ 1060 nm | 10 V/mW @ 1550 nm e | |
MONITOR Output Voltage Swing |
10 V Max, High Z Load | 1.5 V Max for 50 Ω Load 10 V Max (Linear Over 0 to 5 V) for High-Z Load |
||||
Overall Output Voltage Noise | <0.65 mV RMS | <0.6 mV RMS | ||||
DC Offset | < ±2 mV | < ±5 mV | ||||
General | ||||||
Electrical Outputs | SMA | |||||
Included DC Power Supply g | ±12 V @ 250 mA (100/120/230 VAC, 50 - 60 Hz, Switchable) |
|||||
Operating Temperature Range h | 0 °C to 40 °C | |||||
Storage Temperature Range | -40 °C to 70 °C | |||||
Dimensions (W x H x D) | 85 mm x 80 mm x 30 mm (3.35" x 3.15" x 1.18") |
76.7 mm x 60.0 mm x 20.0 mm (3.02" x 2.36" x 0.79") |
||||
Weight | 0.35 kg | 0.13 kg |
Note:
For the PDB480C-AC, PDB481C-AC, and PDB482C-AC detectors the RF output signal must not exceed the RF Output Power at 1 dB Compression, which is the point at which the amplified signal at 1 GHz is compressed by 1 dB. Above this value, the amplified signal will become non-linear and begin to saturate. The RF output voltage at this saturation point can be calculated using the following formula:
where R is the load impedance (50 Ω), P 0 is defined as 1 mW, and L ( dBm ) is the power level in dBm. Using the specified RF Output Power at 1 dB Compression of 16.5 dBm, this yields the following "maximum" output voltage:
Operation of Balanced Detectors with Bandwidths up to 500 MHz
Thorlabs' Balanced Amplified Photodetectors consist of two well-matched photodiodes and an ultra-low noise, high-speed transimpedance amplifier (TIA) that generates an output voltage (RF OUTPUT) proportional to the difference between the photocurrents in the two photodiodes (i.e., the two optical input signals). Additionally, the unit has two fast monitor outputs (MONITOR+ and MONITOR-) to observe the optical input power levels on each photodiode separately. These outputs are low frequency outputs and cannot be used to measure an RF modulation on the signal. The block diagram applies to our balanced detectors with bandwidths up to 500 MHz.
Operation of the PDB480C-AC, PDB481C-AC, and PDB482C-AC Balanced Detectors
The PDB48xC-AC Balanced Amplified Photodetectors use the same basic operating principles as described above and have the following additional features. The TIA's ultra-low distortion output stage supports up to a 2 Vp-p A/D card input range. The two fast monitor outputs can be used to measure not only the individual optical input power levels, but also low frequency modulated signals up to 3 MHz (Item #s PDB480C-AC and PDB481C-AC) or 2.5 MHz (Item # PDB482C-AC). To enable the much higher frequency of these detectors, the photodiodes are connected to the inputs with length-matched fibers, only AC-coupled versions are offered, and capacitors are added to the circuit (as shown below).
Pin Diagrams for Balanced Amplified Photodetectors
Monitor +/-
SMA Female
Maximum Voltage is +10 V for Hi-Z & +1.5 V into 50 Ω loads.
RF Output
SMA Female
For the PDB415, PDB425, PDB440, PDB465, and PDB835 photodetectors, the maximum RF OUTPUT voltage is ±3.6 V for Hi-Z & ±1.8 V into 50 Ω loads.
The RF OUTPUT CW saturation power is 420 µW @ 1550 nm and 530 µW at 1060 nm for the PDB470C and PDB471C, respectively.
For the PDB48xC-AC photodetectors, the RF OUTPUT Power at 1 dB compression into 50 Ω loads is +16.5 dBm (Min), +18 dBm (Typical)
PDB Male (Power Cables)
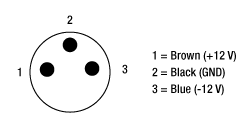
PDB Female (Photodetector)
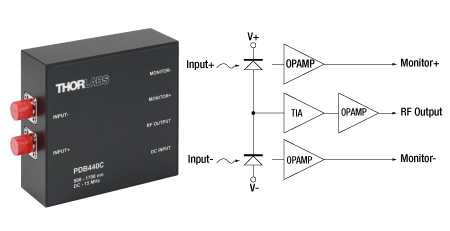
Click to Enlarge
Figure 1. Block Diagram for Thorlabs' Balanced Detectors up to 15 MHz
Application Idea: Doppler LIDAR with Balanced Detector
We demonstrate how to build a Doppler LIDAR (Light Detection and Ranging) system with readily available components manufactured by Thorlabs. The main component is our high-gain balanced photodetector, Item # PDB440C. Balanced detectors are commonly used in applications with high noise levels, which lead to small Signal-to-Noise ratios (SNR).
LIDAR is an ideal application for balanced detectors because they offer common-mode rejection and the usage of both output arms from the 50/50 coupler (described below), which together improve the SNR. Common-mode rejection reduces common-mode noise (for PDB440C, by >25 dB), while using both outputs from the coupler, instead of only one, results in higher signal levels of 3 dB.
The balanced detector consists of two well-matched photodiodes and an ultra-low noise, high-speed transimpedance amplifier (TIA) that generates an output voltage (RF OUTPUT) proportional to the difference between the photocurrents in the two photodiodes.
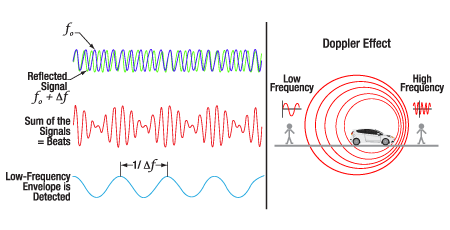
Click to Enlarge
Figure 2. Generation of a beat frequency (left) and principle of the Doppler effect (right).
Theory of Doppler LIDAR
The optical frequency of electromagnetic waves is shifted when reflected from an object in motion. This phenomenon is generally known as the Doppler effect, or, equivalently, as a Doppler shift. When the detector is at a fixed position and the object is moving towards the detector with velocity v, then the Doppler shift Δf can be described as:
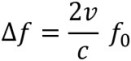
Where:
c: Speed of Light (m/s)
f0: Optical Frequency Emitted by Light Source (Hz)

f1: Optical Frequency of Light Reflected from Object (Hz)
In the typical wavelength ranges of our balanced detectors, optical frequencies lie in the THz regime, which are too fast to record directly with photodiodes. On the other hand, consider the following example:

This example shows that for velocities < 0.75 m/s and an optical frequency of 200 THz, which corresponds to a 1500 nm wavelength, the Doppler shift Δf will be in the kHz regime, meaning that the beat frequency from the reference signal (f0) and the Doppler shifted signal (f1) is detectable with standard photodiodes. The detection of two superimposed signals of different frequencies is known as heterodyne detection.
Setup and Principle
The basic components are a fiber-coupled light source with driver, a fiber coupler with a 99/1 coupling ratio, a fiber coupler with a 50/50 coupling ratio, a fiber circulator, a fiber collimator, a balanced detector, an oscilloscope with Fast Fourier Transform capability, and an object moving with velocity v.
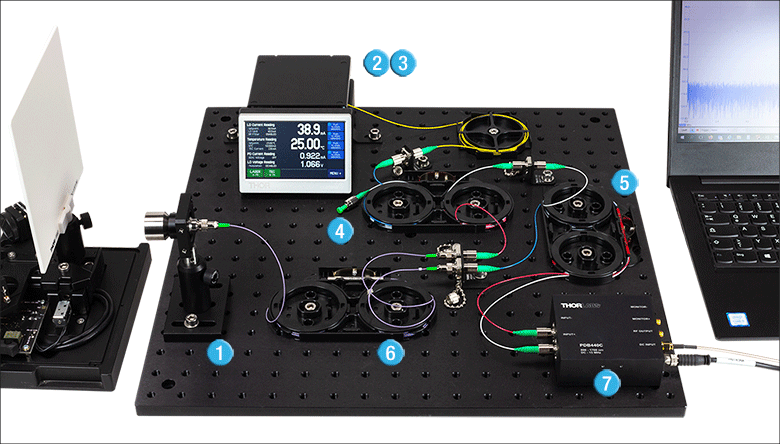
Click to Enlarge
Figure 3. Doppler LIDAR Setup on a Breadboard
Components | Details | |
---|---|---|
1 | Collimator | F810APC-1550 |
2 | Light Source | LP1550-SAD2 DFB Laser with Center Wavelength of 1550.1 nm |
3 | LD & TEC Driver | CLD1010LP Compact Laser Diode/Temperature Controller |
4 | Fiber Coupler (99/1) | TN1550R1A2 |
5 | Fiber Coupler (50/50) | TN1550R5A2 |
6 | Fiber Circulator | 6015-3-APC |
7 | Detector | PDB440C Balanced Photodetector (CMRR > 35 dB; Gain 51 x 103 V/W) |
Analyzer | Standard Oscilloscope (with 500 kHz Fast Fourier Transform) or Spectrum Analyzer | |
Object | EDU-VS1/M Viewing Screen, Mounted on DDSM100/M Linear Stage |
Light emitted by the LP1550-SAD2 DFB Laser is split by a 99/1 fiber coupler. The 99% arm propagates to the fiber circulator, which directs the light to the fiber collimator for coupling into free space. The collimated beam points towards a moving object with velocity v, and the reflected light is coupled into the fiber. On the way back, the circulator guides the light to the 50/50 fiber coupler, whose other input is the 1% arm of the 99/1 coupler. Both outputs from the 50/50 coupler therefore contain mixed optical signals, which are detected by the PDB440C balanced detector.
Measurement and Results
The linear stage is set to a velocity of 250 mm/s. The resulting signal is a sharp peak with an SNR of more than 10 dB. The velocity is calculated as follows:

The accuracy of the velocity of the linear translation stage has an observed deviation of 9 mm/s (3.5%), which is within the expected range of the configuration.
The object is placed on the DDSM100/M linear translation stage, which has a 100 mm travel range. While moving the object, the velocity is non-constant for an unknown distance, because the linear stage has limited acceleration. Thus, the signal's frequency varies while the object travels from 0 mm to 100 mm. The time duration for one measurement at the analyzer, which is an oscilloscope with fast Fourier transform capability, is some tens of milliseconds, so we do not observe continuous movement of the signal, but rather jumps with non-constant intervals. Therefore, we used the maximum peak hold function to evaluate the correlation of the frequency to the maximum velocity of the object. This function captures the peak with highest amplitude during one frequency scan.
This application idea shows a generic configuration and demonstration of a Doppler LIDAR with Thorlabs components. Please contact techsupport@thorlabs.com for questions and comments regarding the application idea or your application.
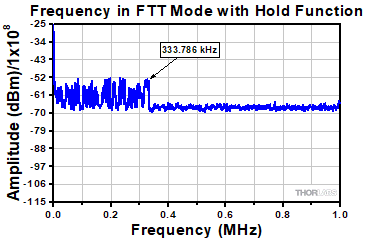
Click to Enlarge
Figure 6. Measurement of the frequency Δf in FFT mode with the oscilloscope max peak hold function.
Posted Comments: | |
user
 (posted 2024-07-31 12:51:29.13) For the PDB835 on the specs tab, RF OUTPUT CW Saturation Power should have units of microWatts, right? jjadvani
 (posted 2024-08-01 02:46:18.0) Dear user, Thank you for providing feedback. The PDB835 has an RF output CW saturation power of 360 µW at 1550 nm. There is a typo in the spec table, which we will fix soon. Jerard Vincent Rubio Ang
 (posted 2024-05-17 19:28:22.223) What size screws are used on the FC adapter? We lost some of the screws for ours. jjadvani
 (posted 2024-05-17 08:30:39.0) Dear Jerard, Thank you for your feedback. The size of the screw for FC fiber input holder is M2 x 4 Allen screw. Jaeyoon Ko
 (posted 2024-03-06 15:58:04.61) Dear Thorlabs Team, Hello! I'm recently recieved your PDB425A-AC and tried to use for balanced photodetection in pump-probe setup. I am using femtosecond 520 nm laser. My probe beam reflects from the sample and enters Input + and planning to induce sampled probe beam to Input - port.
However, there are some phase difference between two inputs. When I induce probe beam and view through oscilloscope(triggered by laser source internal oscillator), there are 5 ns of phase difference. Is this normal? dpossin
 (posted 2024-03-06 08:40:59.0) Dear Yaeyoon,
Thank you for your feedback. A possible reason for a phase difference could be that the optical path lengths are not equal. You can view the optical path length difference vs frequency shift on the PDB manual in section 3.7. One method in order to compensate for that is to use an optical delay line.
I reach out to you directly to discuss your application in more detail.
Best regards,
Dominik Kye Kwanuk
 (posted 2024-02-04 22:54:59.377) Hello. I'm interested in Thorlabs's doppler lidar detector, PDB series.
I was looking for some doppler lidar publications, and many publications used PDB430C-AC detector from Thorlabs. but PDB430C-AC is obsoleted, and it seems that PDB435C-AC has simillar spec.
My question is that,
Is PDB435C-AC an upgraded version of product PDB430C-AC? or are they just seperated products?
Thanks hkarpenko
 (posted 2024-02-07 02:54:18.0) Dear customer,
thank you for your feedback. We use a revised photodiode of the same model for this new balanced detector. The specifications are very similar to the obsolete version, as you already mention. I will contact you directly to discuss your application in detail and help you choosing the correct detector. Nikita Kikilich
 (posted 2023-12-12 09:49:44.517) Hello! We are using PDB470C-AC for measurement application. We are faced with a problem that is very similar to restoring a photodetector after a strong signal. Could you give us additional information about the model of the photodetector (the PIN diode itself) or at least its capacity, saturation threshold, and so on. dpossin
 (posted 2023-12-13 09:20:55.0) Dear Nikita,
Thank you for your feedback. I reach out to you in order to provide the requested details. dpossin
 (posted 2023-12-13 09:20:55.0) Dear Nikita,
Thank you for your feedback. I reach out to you in order to provide the requested details. Markus Schmit
 (posted 2023-10-07 13:23:45.87) Dear Thorlabs team! Thank you very much for your explanation about Homodyne detectors. I have a PDB425C-AC detector and would like to ask you about laser power applied to this detector in my setup. According to its datasheet, it's said that to avoid saturation in Monitors and to prevent nonlinearities in RF output, the input power should be less than 1mW and 4W/cm2, respectively. Are they DC power or peak power?
Many thanks and best regards, Markus. hkarpenko
 (posted 2023-10-12 04:09:39.0) Dear Markus,
thank you for your kind feedback. In general these values are tested for a CW input. We have not tested the saturation power for pulsed sources, thus I would expect the threshold value to be a little higher. For your application I would recommend to start with a value below 1mW peak power and slightly increase it until you see saturation on the detector. That way you will avoid damage to the amplifier. The maximum damage threshold of the photodiode is 20mW (CW and peak power). Exceeding this value will permanently damage the detector. Semyon Vasilchenko
 (posted 2023-05-29 10:14:21.317) Dear Sir or Madam, Monitor channel Transimpedance Gain is not given in specs. Do I properly calculate it for PDB450C as 10000 V/W since Max Responsivity for a photodiode is given as 1.0 A/W and Conversion gain is 10 V/mW @ 1550 nm? If I'm wrong please tell me Monitor Output Transimpedance Gain. hkarpenko
 (posted 2023-05-31 06:05:40.0) Dear customer,
thank you for your feedback. Indeed, you did calculate it correctly by using the responsivity a conversion gain CG. The TIA gain is 10^4 V/A of the monitor port. William P
 (posted 2023-03-07 15:32:37.563) Dear Thorlabs,
I very much appreciate your explanations and application ideas. Regarding Doppler lidar, I have a simple (maybe naive) question: I understand that using a balanced detector can significantly reduce common noise, but does the useful beat signal also decrease due to the difference in photocurrents between the two photodiodes? Thank you in advance.
William hkarpenko
 (posted 2023-03-09 11:05:27.0) Dear William,
thank you very much for your kind feedback.
The beat signal will not be decreased, since it will only be present on one of the two photodiodes. I will contact you directly to discuss this further with you. Vladimir Zenin
 (posted 2023-02-08 15:27:29.193) Dear Thorlabs,
I would like to ask your advice regarding the noise. In my setup I need to measure small modulations on top of a large nearly-DC signal, therefore I have kind of lock-in setup. Here is what I noticed: even when I have no modulations, and light comes directly to the detector, there is a signal (noise) produced by lock-in. And this noise is proportional to the optical power. Is this noise somehow related to NEP, or is it a different specification of the detector? I always though NEP is a noise with no optical power hitting the detector. Also, about my case, I have balanced detectors PDB450A and PDB450C, and I can verify that the noise I see is not from the laser (the noise is present in RF output, and doesn't change after unbalancing the detector). If you have any suggestions for a different detector for my setup, it is more than welcome :) Thank you in advance! dpossin
 (posted 2023-02-14 04:47:36.0) Dear Vladimir,
Thank you for your feedback. I am looking into this. I am reaching out to you directly in order to discuss it in more detail. Vladimir Zenin
 (posted 2023-01-11 17:02:31.933) Dear Thorlabs,
My question is about switchable-gain detector PDB450A-AC. In specs it says CW Saturation Power RF Output 9 mW, which, probably, produces 10V at RF output at lowest gain. However, and this power Monitors will be way beyond saturation (their gain is 10 V/mW, and max 10V output). I am wondering, is it ok to operate the detector with saturated monitors, but when RF output is below saturation (i.e., with 9 mW of optical power)? If not, what is the upper limit for normal operation? Thank you in advance!
P.S. I have both PDB450A and PDB450C detectors - very satisfied with them! hkarpenko
 (posted 2023-01-12 07:59:24.0) Dear Vladimir,
thank you for your kind feedback. You are correct, the CW saturation power of the RF output is 9mW, while the saturation power of the monitor ports is only 1mW according to the spec sheet.
I will contact you directly to discuss this issue further with you. user
 (posted 2022-12-28 17:02:50.843) When I connect the Monitor output+ to an oscilloscope for 50 ohm load and connect the RF output for 50 ohm load also, the amplitude of RF output was about 15-times-larger than Monitor output's one.
The Conversion Gain of RF and Monitor output for 50 ohm load are 25V/mW and 10V/mW, respectively, so I expected that the output of RF becomes 2.5-times-larger than that of Monitor.
What causes this? dpossin
 (posted 2022-12-28 06:41:21.0) Dear Hiroki,
Thank you for your feedback. The main difference between the RF output and the monitor output is the bandwidth. For the PDB415C the bandwidth of the RF output is 100MHz while the bandwidth for the monitor outputs is limited to 1MHz. The main purpose of the monitor outputs is alignment. Since they show crosstalk too they are not well suited for the actual balanced measurement. I am reaching out to you in order to dig in in more detail. Megha Jain
 (posted 2022-09-29 10:57:42.717) I want to know the rise time of detector. hkarpenko
 (posted 2022-09-29 08:36:45.0) Dear Megha,
thank you for your feedback.
The risetime of a detector can be calculated from the bandwidth according to this formula: risetime = 0.35/bandwidth. The PDB425A has a maximum bandwidth of 75MHz, which corresponds to a risetime of 4.7ns. I contact you directly to discuss this further with you. Felix B
 (posted 2022-02-23 07:08:29.95) Dear Sir or Madam,
I have the PDB450C detector and have unscrewed the fibre holders. Now I would like to attach a (self constructed) mount for a lens (e.g. LA1540-C) to the attached four threads. For this I need the distance of the active surface of the photodiodes to a reference plane. Could you tell me this distance? Unfortunately, I did not find this information in the data sheet.
It would also be interesting to know the tolerances with which the photodiodes are mounted.
Best regards, Felix dpossin
 (posted 2022-02-23 10:50:04.0) Dear Felix,
Thank you for your feedback. The distance between the coverglass of the photodiode and the fiber tip is 0.4 +/- 0.05mm. I am reaching out to you to discuss this in more detail. Jameel Ali
 (posted 2021-12-14 06:49:39.44) Dear Sir/Madam,
We have an optical fiber sensing experiment aimed to sense vibration signals or events. In our experiment, se send a laser at 1550nm through the fiber, and that laser wave will be picking the phase /amplitude variations in the fiber. And at the end of the fiber, we want to use a photodetector that can detect the signals.
Our vibration signal has low bandwidth (few Hz - tens of kHz). In addition, the received optical power in our experimental setup is very low ( in the range of -xx.x dBm). Therefore, we would like you please to recommend a suitable photodiode/photoreceiver model to help us in our application. soswald
 (posted 2021-12-17 06:53:07.0) Dear Jameel,
thank you for your feedback. I have reached out to you directly to discuss your application in more detail. Aleksey Lopatin
 (posted 2021-09-29 10:21:12.447) Dear Sir/Madam,
How do you define SMRR for PDB425A? (I've bought one already).
As SMRR=10log(V1/V2) or SMRR=20log(V1/V2). Speaking about voltage output, it should be SMRR=20log(V1/V2). However, in some literature, related to balanced photodetectors, one can find definition SMRR=10log(I1/I2).
What is your SMRR definition?
Regards,
Aleksey dpossin
 (posted 2021-10-06 08:25:27.0) Dear Aleksey,
Thank you for your feedback. We measure the CMRR with a network analyzer. Therefore we use a laser modulated by the network analyzer and splitted into two path with a 3dB fusion coupler such that the path length difference is as small as possible. Both branches of the coupler output are connected to the input ports of the PDB. On the other side the RF output of the PDB is connected to the measurement port of the networt analyzer. The difference between both inputs and the balanced signal at the 3 dB frequency means the CMRR value. Please also see a more detailed explanation in the PDB manual: https://www.thorlabs.de/thorproduct.cfm?partnumber=PDB425A user
 (posted 2021-08-31 07:24:27.86) The typical detector responsivity curve shows that the detector is still responsive to light at 1600 nm, but why the working wavelength (shaded area) can only reach 1400 nm dpossin
 (posted 2021-09-07 10:13:36.0) Dear customer,
Thank you for your feedback. The graph shows the responsivity of the photodiodes inside the PDB482C-AC but the usable working range is limited by the fiber which connects the fiberports to the photodiodes. The highlighted intersection represents the working range. I am reaching out to you to discuss this further. David shoala
 (posted 2021-08-09 13:16:55.207) Hello
I'm David
I hope you are well
We have already one Balanced Detector from your company( model: PDB470c )
Unfortunately Carelessly we were working with a 20mW CW laser for 1 minute permanently after that one of two detectors doesn’t have any response on an oscilloscope.
Is that detector is destroyed ??
If yes but I have a question about photodiode
Is this photodiode replaceable?
How to repair this balanced detector
Best regards
David dpossin
 (posted 2021-08-09 07:35:47.0) Dear David,
Thank you for your feedback. Since the damage threshold has been exceeded most probably the photodiode which has been exposed is damaged now. However we can replace the damaged photodiode via RMA. I am reaching out to you in order to provide further details on the process. Minsuk Lee
 (posted 2021-05-24 18:36:11.37) We are currently using a PDB465C for an 1300nm SSOCT application.
We need a response time to correctly select k-trigger delay time.
Can you tell me what the response time is? MKiess
 (posted 2021-05-27 09:07:39.0) This is a response from Michael at Thorlabs. Thank you very much for your inquiry. The rise time of the balanced output signal can be calculated from the signal bandwidth using this formula: Rise time = 0.35 / Bandwidth. The bandwidth of the PDB465C is 200 MHz. This corresponds to a rise time of 1.75 ns I will contact you directly to provide further assistance. 陈 潜源
 (posted 2021-05-21 11:37:03.35) 我们有个在您们公司买的类似这款探测器,S/N.:M00319347。现在我想要他的说明书和规格参数,请问您们能发送到我的邮箱吗? YLohia
 (posted 2021-05-24 10:37:44.0) Thank you for contacting Thorlabs. We will reach out to you directly. Seth Erickson
 (posted 2020-10-26 14:52:00.707) On the PDB415A, the two photodiodes are mounted 20 mm apart. While this is fine for fiber coupled operation, it creates a problem for free space coupling. Since we use 1 inch optics with 1/2" posts & post holders, it is actually impossible to get proper lens alignment, since having the center of the lenses 20 mm apart results in overlapping lenses. Since this is a common lab setup, it seems silly that the photodiodes are 20 mm apart instead of 30-35 mm, which would provide 5-10 mm separation between lenses, allowing room for pedestal mounting. Might be a silly nitpick, but thought you might appreciate the feedback. Cheers MKiess
 (posted 2020-10-27 10:26:35.0) Dear Seth, Thank you very much for this feedback. This is not a silly nitpick. Such feedback helps us to constantly improve our products. Even though the PDB415A is mainly designed for fiber applications, it is also suitable for free space applications due to the possible removal of the FC adapter. Therefore, it is correct that the distance is not ideal for a free space application with the standard sizes, 1/2" or 1" optics. Thank you very much for this information. For applications with larger optics the detector you can find under the following link would be more suitable:
https://www.thorlabs.de/newgrouppage9.cfm?objectgroup_id=1299&pn=PDB210A Qiangzhou Rong
 (posted 2020-06-17 09:13:10.84) Dear Mr/Mrs/Miss,
I am postdoc at Duke University. We bought a PDB425C-AC detector recently. The noise from the RF port is larger than 100 mV without any light input. It is too large to be used in experiment. Is it correct or something rong in operation make it so large? Could you give me some comments as soon as possible? Urgently waiting for kind help! Thank you very much!
Best regards,
Qiangzhou Rong MKiess
 (posted 2020-06-19 07:31:15.0) This is a response from Michael at Thorlabs. Thank you for the inquiry.A noise of more than 100mV is too high for this detector, if used properly. I have contacted you directly to discuss your application and find a solution together with you. wu nigel
 (posted 2020-02-05 17:39:41.227) please tell me Balanced detector life MKiess
 (posted 2020-02-06 06:18:35.0) This is a response from Micael at Thorlabs. Thank you for the inquiry. I have contacted you directly to discuss the influences on the lifetime of the detector in relation to your application. Kristof Reynkens
 (posted 2019-06-27 05:02:47.707) I wondered if the PDB450A(-AC) can be cooled and how the NEP would change vs temperature. dpossin
 (posted 2019-06-28 09:57:23.0) Hello Kristof,
Thank you for your Feedback. In general the NEP will decrease when you lower the temperature. The NEP with respect to wavelength can be calculated the following way: NEP(lambda)=(R_max/R(lambda))x NEP_min.
There R_max is the maximum responsivity, R(lambda) is the responsivity with respect to the wavelenght and NEP_min is the NEP value which is specified on the homepage.
The detector can be cooled but you will need to build up the cooling circuit yourself.
I will reach out directly to you to support you with temperature related data on the photodiode itself. Jonathan Doorn
 (posted 2019-04-01 18:34:40.513) I am running some noise experiments with this device, the PDB450A, and I would like to know more about the noise characteristics of this device. What opamps does this device use? swick
 (posted 2019-04-05 03:46:29.0) This is a response from Sebastian at Thorlabs. Thank you for the inquiry. In the manual we show graphs with typical spectral noise for PDB450A at each gain setting. I contacted you directly to provide further assistance. zenin
 (posted 2019-02-20 10:03:49.533) Hi,
Currently I am using PDB450C and PDB450A-AC. I found that AC-coupled detection is better for my setup, so I am wondering, is it possible to upgrade my PDB450C to AC-coupled version? If yes, what is time scale and price?
Additionally, I would like to give you two proposals for making it better:
1) It would be nice to have a single device with on-off switch for AC-coupling. Then people like me would not make trials & errors on which one to buy.
2) The holder for post-mounting (with 4 holes to attach to detector) is rather disappointing. It seems to be made of plastic, and one cannot screw it tight. You see, I am using both VIS and NIR detectors for free-space beams, and I wanted to attach each BD to magnetic mount in order to have fast switch, when I change the source. The light is focused by two parabolic mirrors, and there are two precision translation 3D stages, so I wanted to not buy and build separate sets of those for each BD. But mounting of BD to magnetic mount was a nightmare: 4 holes in BD are not standart-size (I would prefer separation of 2.5 cm or separation like for the cage system). I hope that in future you will make this mount better and compatible with standart Thorlabs dimensions.
Best,
Vladimir nreusch
 (posted 2019-02-27 01:55:14.0) This is a response from Nicola at Thorlabs. Thank you for your good suggestions! We will take them into account in the future. We can also offer to upgrade the PDB to the -AC version. I will contact you directly with further information. Carsten.Kuehn
 (posted 2018-01-08 18:36:56.437) In your Datasheet the PDB415C is speced for tempertures upto 40degC.
What will happen when the amplifier is used at higer temperatures?
Is there a high temperature version of this amplifier, which is able to operate at 75degC ambient.
Thanks in advance! swick
 (posted 2018-01-12 03:21:02.0) This is a response from Sebastian at Thorlabs. Thank you for the inquiry. All specified parameters for PDB415C can not be guaranteed when used outside of operating temperature range. At 75°C the detector should still work but performance will be worse. We do not offer high-temperature versions for this detector. gregory.gaeumann
 (posted 2017-06-23 09:50:38.3) What's the rise time of the balanced output signal of PDB450A(-AC)? wskopalik
 (posted 2017-06-23 10:17:58.0) This is a response from Wolfgang at Thorlabs. Thank you very much for your inquiry.
The rise time of the balanced output signal can be calculated from the signal bandwidth using this formula: Rise time = 0.35 / Bandwidth.
The bandwidth of the PDB450A / PDB450A-AC is in the range of 0.1 - 150 MHz depending on the gain setting. This corresponds to rise times of 3.5 µs at 0.1 MHz and 2.3 ns at 150 MHz.
I will contact you directly to provide further assistance. john_noriega
 (posted 2016-11-22 15:12:31.733) Can you please clarify the spec "CW Saturation Power RF-Output" Is that the optical power that will saturate the RF output, or is that the optical power that saturates the photodiode, thus limiting the RF output? Or am I missing some other interpretation? swick
 (posted 2016-11-23 04:56:10.0) This is a response from Sebastian at Thorlabs. Thank you very much for the inquiry.
The parameter "CW Saturation Power RF-Output" is referred the power difference between the optical inputs. This value must not be exceeded to prevent saturation of the balanced amplifier, which would cause nonlinear behavior.
Saturation of the Monitor outputs will occur at optical input power levels greater than 1 mW.
You can find this explanation in the manual at Chapter 3. mahldyla
 (posted 2015-02-27 11:42:57.35) To whom it may concern: Could you explain how the plots of "spectral noise" and "frequency response" are measured for each of the balanced detectors? The reason that I ask is that, with balanced inputs, the output should be shot noise, which is white. I would expect both plots to be identical then, if I am not misunderstanding anything. Also, is there a way to flatten the frequency response of the detector? tschalk
 (posted 2015-03-03 10:15:39.0) This is a response from Thomas at Thorlabs. Thank you very much for your inquiry. Please note that the measurement of "spectral noise" and "frequency response" is explained in the manual of the PDBs: http://www.thorlabs.de/thorcat/21600/PDB450C-AC-Manual.pdf. You can find the information you are looking for at section 3.6 CMRR and Frequency Response and at section 5 Appendix Typical noise spectra. Unfortunately, it is not possible to flatten the frequency response. I will contact you directly with more detailed information. kiwa
 (posted 2015-01-08 04:02:02.833) What is the rise and fall time of the PDB410A?
I want to use it together with Maitai Ti:sapphire oscilator at 42 MHz together with boxcar which will sellect one pulse at 1kHz rate for THz detection via balanced detection.
I'm wondering if 100 MHz diodes are fast enough, or shall I go for 350 MHz?
It is a free space optics setup so it's better if photodiodes have large area. shallwig
 (posted 2015-01-09 03:40:54.0) This is a response from Stefan at Thorlabs. Thank you very much for your inquiry. You can calculate the rise time of the PDB410A by using the specified 3dB bandwidth and the following formula: f(3dB)=0.35/rise time. This gives you a rise time of 3.5ns. This limitation comes from the built in amplifier. I will contact you directly to discuss your application in detail and to check which PDB meets your requirements best. bpursley
 (posted 2014-12-29 12:40:43.14) To whom it may concern:
Do you have a model (or could suggest one) for the frequency response of the various gain settings on the PDB450A? In particular, the 10^3 and 10^4 gain settings? Thanks in advance! shallwig
 (posted 2015-01-07 04:59:03.0) This is a response from Stefan at Thorlabs. Thank you very much for your inquiry. We specify the frequency response for various gain settings in the spec sheet of the PDB450A as follows:
RF OUTPUT Bandwidth (-3 dB): DC - 150 / 45 / 4 / 0.3 / 0.1 MHz
RF OUTPUT Transimpedance Gain: 10^3 / 10^4 / 10^5 / 10^6 / 10^7 V/A
So for 10^3 and 10^4 gain settings the 3dB bandwidth is specified with DC-150MHz and DC-45MHz . In the manual http://www.thorlabs.com/thorcat/21600/PDB450A-Manual.pdf on page 31 you can also find a curve showing the frequency versus amplitude for the different gain settings.
I will contact you directly to discuss your application in detail and to check if you need further information. bdada
 (posted 2011-12-29 11:27:00.0) Response from Buki at Thorlabs:
To keep everything free-space, I would recommend post mounting two mirrors to an optical table. To minimize space requirements, one of the mirrors can be D shaped. We have sent you a schematic describing the set up.
Unfortunately there is not an easy way to mount a lens directly to the balanced detector box.
Please contact TechSupport@thorlabs.com if you have any questions. boris.povazay
 (posted 2011-12-27 16:24:59.0) Dear Thorlabs team!
You mention that free-space coupling onto the detectors is possible with by dismounting the FC-connectors. However - it is unclear how to couple into that 0.8-0.3mm wide detectors. Especially since they are so close together it is extremely challenging to hit them with a focussed beam. Do you have any suggestions up your sleeve on how to mount pair of lenses with x-y-z positioning capability to the detector box (rather than independently in front of the device)?
Many thanks and best regards,
Boris jvigroux
 (posted 2011-12-05 09:53:00.0) A response from Julien at Thorlabs: the distance between the photodiode chip and the ferrule of the fiber is between 600µm and 1.1mm. This range is the sum of the mechanical tolerances given by the photodiode manufacturer and ours. user
 (posted 2011-11-30 15:31:01.0) A response from Tyler at Thorlabs to Sergey: The diameter of the photodiodes is 0.8 mm. We are looking into the distance between the fiber tip and the photodiode. We will contact you with this information and help you determine an appropriate fiber. Sergey.L.Vinogradov
 (posted 2011-11-29 01:14:37.0) Dear support staff,
I try to use PDB450A in Dynamic Light Scattering Spectroscopy at 633 nm with various MM fiber core diameter ranging from 50 to 400 um. Regretfully, I did not find any information on alignment of FC receptacle to photodiode except some general remark:
"In general, multi-mode fiber at the input can be used, but in this case the light beam
spot diameter exceeds detector’s active area, which results in a reduced output
signal as well."
Nevertheless, I assume that some large core fiber with low NA could be coupled to photodiode aperture without losses.
Please, let me see some mechanical drawing related to that, at least clarifying the distance between receptacle and photodiode. I would also appreciate your advises on appropriate combination of NA and core diameter providing lossless coupling.
Thanks in advance,
Sergey Vinogradov jvigroux
 (posted 2011-06-06 08:17:00.0) A response from Julien at Thorlabs: Dear Claudius, the bandwidth of the monitor outputs is unfortunately limited by the amplifier that is used for those. An upgrade up to 100MHz or higher is of course technically possible but would imply a large amount of modification and would probably driver the price up substantially. Should you be interested anyways, please contact our technical support at techsupport@thorlabs.com weimann
 (posted 2011-06-03 06:59:44.0) Dear Sir or Madam,
Is there the possibility to get a balanced detector with an even faster monitor output?
For a current research project a balanced detector with the possbibility to read out a ~100 MHz (or faster) signal from the single PDs would be really helpful.
Yours sincerely,
Claudius Weimann. |

Specificationsa | ||||
---|---|---|---|---|
Item # | PDB450A(-AC) | PDB450C(-AC) | ||
Detector Type | Si/PIN | InGaAs/PIN | ||
Wavelength Range | 320 - 1000 nm | 800 - 1700 nm | ||
Max Responsivity (Typical) | 0.53 A/W | 1.0 A/W | ||
Active Detector Diameter | 0.8 mm | 0.3 mm | ||
Bandwidth (3 dB) | DC - 150, 45, 4, 0.3, 0.1 MHz AC Coupled (-AC Suffix): 100 Hz - 150, 45, 4, 0.3, 0.1 MHz |
|||
Common Mode Rejection Ratio |
>25 dB | |||
Transimpedance Gainb | 103, 104, 105, 106, 107 V/A | |||
Minimum NEP | DC - 0.1 MHz: 1.4 pW/Hz1/2 DC - 0.3 MHz: 1.1 pW/Hz1/2 DC - 4.0 MHz: 3.3 pW/Hz1/2 DC - 45 MHz: 28.9 pW/Hz1/2 DC - 150 MHz: 123 pW/Hz1/2 |
DC - 0.1 MHz: 0.7 pW/Hz1/2 DC - 0.3 MHz: 0.5 pW/Hz1/2 DC - 4.0 MHz: 1.55 pW/Hz1/2 DC - 45 MHz: 14.9 pW/Hz1/2 DC - 150 MHz: 68.6 pW/Hz1/2 |
||
Optical Inputs | FC/PC or FC/APC Compatible (Removable Adapter) | |||
Monitor Output Bandwidth | DC - 1 MHz |

Specificationsa | ||||
---|---|---|---|---|
Item # | PDB440A(-AC) | PDB440C(-AC) | ||
Detector Type | Si/PIN | InGaAs/PIN | ||
Wavelength Range | 320 - 1000 nm | 800 - 1700 nm | ||
Max Responsivity (Typical) | 0.53 A/W | 1.0 A/W | ||
Active Detector Diameter | 0.8 mm | 0.3 mm | ||
Bandwidth (3 dB) | DC - 15 MHz AC Coupled (-AC Suffix): 100 Hz - 15 MHz |
|||
Common Mode Rejection Ratio | >35 dB | |||
Transimpedance Gainb | 51 x 103 V/A | |||
Minimum NEP | 6.9 pW/Hz1/2 (DC - 15 MHz) |
3.9 pW/Hz1/2 (DC - 15 MHz) |
||
Optical Inputs | FC/PC or FC/APC Compatible (Removable Adapter) | |||
Monitor Output Bandwidth | DC - 1 MHz |

Specificationsa | ||
---|---|---|
Item # | PDB425A(-AC) | PDB425C(-AC) |
Detector Type | Si/PIN | InGaAs/PIN |
Wavelength Range | 320 - 1000 nm | 800 - 1700 nm |
Max Responsivity (Typical) | 0.53 A/W | 1.0 A/W |
Active Detector Diameter | 0.8 mm | 0.3 mm |
Bandwidth (3 dB) | DC - 75 MHz AC Coupled (-AC Suffix): 100 Hz - 75 MHz |
|
Common Mode Rejection Ratio | >35 dB | |
Transimpedance Gainb | 250 x 103 V/A | |
Minimum NEP | 9.5 pW/Hz1/2 (DC - 75 MHz) |
5.2 pW/Hz1/2 (DC - 75 MHz) |
Optical Inputs | FC/PC or FC/APC Compatible (Removable Adapter) | |
Monitor Output Bandwidth | DC - 1 MHz |

Specificationsa | ||
---|---|---|
Item # | PDB415A(-AC) | PDB415C(-AC) |
Detector Type | Si/PIN | InGaAs/PIN |
Wavelength Range | 320 - 1000 nm | 800 - 1700 nm |
Max Responsivity (Typical) | 0.53 A/W | 1.0 A/W |
Active Detector Diameter | 0.8 mm | 0.3 mm |
Bandwidth (3 dB) | DC - 100 MHz AC Coupled (-AC Suffix): 100 Hz - 100 MHz |
|
Common Mode Rejection Ratio | >25 dB | |
Transimpedance Gainb | 50 x 103 V/A | |
Minimum NEP | 12.03 pW/Hz1/2 (DC - 100 MHz) |
6.99 pW/Hz1/2 (DC - 100 MHz) |
Optical Inputs | FC/PC or FC/APC Compatible (Removable Adapter) | |
Monitor Output Bandwidth | DC - 1 MHz |

Specificationsa | ||
---|---|---|
Item # | PDB465A(-AC) | PDB465C(-AC) |
Detector Type | Si/PIN | InGaAs/PIN |
Wavelength Range | 320 - 1000 nm | 800 - 1700 nm |
Max Responsivity (Typ.) | 0.50 A/W | 1.0 A/W |
Active Detector Diameter | 0.8 mm | 0.15 mm |
Bandwidth (3 dB) | DC - 200 MHz AC Coupled (-AC Suffix): 100 Hz - 200 MHz |
|
Common Mode Rejection Ratio |
>25 dB | |
Transimpedance Gainb | 30 x 103 V/A | |
Minimum NEP | 22.86 pW/Hz1/2 (DC - 200 MHz) |
8.52 pW/Hz1/2 (DC - 200 MHz) |
Optical Inputs | FC/PC or FC/APC Compatible (Removable Adapter) |
FC/PC or FC/APC Compatible (Adapter is not Removable) |
Monitor Output Bandwidth | DC - 1 MHz |

Specificationsa | ||
---|---|---|
Item # | PDB435A(-AC) | PDB435C(-AC) |
Detector Type | Si/PIN | InGaAs/PIN |
Wavelength Range | 320 - 1000 nm | 800 - 1700 nm |
Max Responsivity (Typ.) | 0.50 A/W | 1.0 A/W |
Active Detector Diameter | 0.4 mm | 0.15 mm |
Bandwidth (3 dB) | DC - 350 MHz AC Coupled (-AC Suffix): 100 Hz - 350 MHz |
|
Common Mode Rejection Ratio |
>20 dB | |
Transimpedance Gainb | 10 x 103 V/A | |
Minimum NEP | 32.3 pW/Hz1/2 (DC - 350 MHz) |
15.28 pW/Hz1/2 (DC - 350 MHz) |
Optical Inputs | FC/PC or FC/APC Compatible (Removable Adapter) |
FC/PC or FC/APC Compatible (Adapter is not Removable) |
Monitor Output Bandwidth | DC - 1 MHz |

Specificationsa | ||
---|---|---|
Item # | PDB471C(-AC) | PDB470C(-AC) |
Operating Wavelength | Optimized for 1060 nm (900 - 1400 nm Range) |
Optimized for 1300 nm (1200 - 1700 nm Range) |
Detector Type | InGaAs/PIN | |
Internal Coupling Fiber | HI1060 | SMF-28e+ |
Responsivity (Typical) | 0.72 A/W @ 1060 nm | 0.9 A/W @ 1550 nm |
Active Detector Diameter | 0.080 mm | 0.075 mm |
Bandwidth (3 dB) | DC - 400 MHz AC Coupled (-AC Suffix): 100 Hz - 400 MHz |
|
Common Mode Rejection Ratio | >25 dB (Typ. >30 dB) |
|
Transimpedance Gainb | 10 x 103 V/A | |
Minimum NEP | 8 pW/Hz1/2 (DC to 100 MHz) | |
Optical Inputs | FC/APC | |
Monitor Output Bandwidth | DC - 3 MHz |
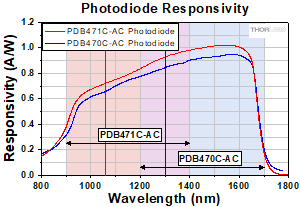
Click to Enlarge
The two vertical lines mark 1060 nm (red) and 1300 nm (blue). The shaded regions indicate the specified wavelength range for each detector.

Specificationsa | |
---|---|
Item # | PDB835C(-AC) |
Detector Type | InGaAs/PIN |
Wavelength Range | 800 - 1700 nm |
Max Responsivity (Typical) | 1.0 A/W @ 1550 nm |
Active Detector Diameter | 0.08 mm |
Bandwidth (3 dB) | DC - 500 MHz AC Coupled (-AC Suffix): 1 kHz - 500 MHz |
Common Mode Rejection Ratio | >25 dB |
Transimpedance Gainb | 10 x 103 V/A |
Minimum NEP | 6.5 pW/Hz1/2 (DC to 500 MHz) |
Optical Inputs | FC/APC |
Monitor Output Bandwidth | DC - 1 MHz |

Specificationsa | ||
---|---|---|
Item # | PDB481C-AC | PDB480C-AC |
Operating Wavelength | Optimized for 1060 nm (900 - 1400 nm Range) |
Optimized for 1300 nm (1200 - 1700 nm Range) |
Detector Type | InGaAs/PIN | |
Internal Coupling Fiber | HI1060 | SMF-28e+ |
Responsivity (Typical) | 0.72 A/W @ 1060 nm | 0.9 A/W @ 1550 nm |
Active Detector Diameter | 0.080 mm | 0.075 mm |
Bandwidth (3 dB) | 30 kHz - 1.0 GHz | 30 kHz - 1.6 GHz |
Common Mode Rejection Ratio |
>25 dB (Typ. >30 dB) |
|
Transimpedance Gainb | 16 x 103 V/A | |
Minimum NEP | 9.0 pW/Hz1/2 (30 kHz to 100 MHz) |
9.3 pW/Hz1/2 (30 kHz to 100 MHz) |
Optical Inputs | FC/APC | |
Monitor Output Bandwidth | DC - 3 MHz |
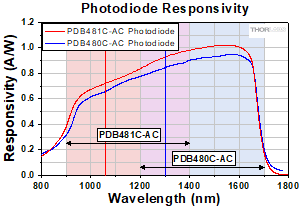
Click to Enlarge
The two vertical lines mark 1060 nm (red) and 1300 nm (blue). The shaded regions indicate the operating wavelength range for each detector.

Specificationsa | |
---|---|
Item # | PDB482C-AC |
Operating Wavelength | Optimized for 1060 nm (900 - 1400 nm Range) |
Detector Type | InGaAs/PIN |
Internal Coupling Fiber | HI1060 |
Responsivity (Typical) | 0.72 A/W @ 1060 nm |
Active Detector Diameter | 0.080 mm |
Bandwidth (3 dB) | 1 MHz - 2.5 GHz |
Common Mode Rejection Ratio | >20 dB (>25 dB Typ.) |
Transimpedance Gainb | 28 x 103 V/A |
Minimum NEP | 12.0 pW/Hz1/2 (1 MHz to 100 MHz) |
Optical Inputs | FC/APC |
Monitor Output Bandwidth | DC - 2.5 MHz |
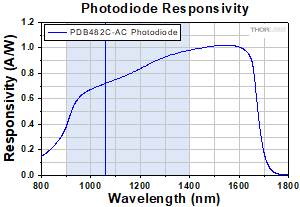
Click to Enlarge
The vertical line marks the detector's optimized wavelength (1060 nm) while the shaded region indicates the operating wavelength range.

- Replacement Power Supply for the Balanced Amplified Photodetectors Sold Above
- ±12 VDC Power Output
- Current Limit Enabling Short Circuit and Overload Protection
- On/Off Switch with LED Indicator
- Switchable AC Input Voltage (100, 120, or 230 VAC)
- 2 m (6.6') Cable with LUMBERG RSMV3 Male Connector
- UL and CE Compliant
The LDS12B ±12 VDC Regulated Linear Power Supply is intended as a replacement for the supply included with our PDB line of balanced photodetectors sold on this page. The cord has three pins: one for ground, one for +12 V, and one for -12 V (see diagram to the right). A region-specific power cord is shipped with the unit based on your location. This power supply can also be used with the PDA series of amplified photodetectors, PMM series of photomultiplier modules, APD series of avalanche photodetectors, and the FSAC autocorrelator for femtosecond lasers.